The Rationale for an Automatic Spectroscopic Telescope
Robotic Telescopes
ASP Conference Series, Vol. 79, 1995
Gregory W. Henry and Joel A. Eaton (eds.)
Joel A. Eaton
Center of Excellence in Information Systems, Tennessee State University, Nashville, TN U.S.A.
Abstract. Computers have changed our lives by making it possible to control large amounts of information effectively. This, in turn, makes the manufactured goods we use cheaper and makes them better. An entirely parallel development is occurring in astronomy, for the same reasons. We discuss the application of computer-based automation to astronomy, specifically to observing, and discuss reasons for extending it to spectroscopy.
1. Introduction
Over the past twenty years there has been a revolution in manufacturing brought on by application of computers to planning and controlling complex operations. This change has been accompanied by a bewildering number of acronyms as well as unjustified hyperbole, but the fact remains that computers have made manufacturers a lot more efficient as well as nimbler, with marked effects on employment. In 1980, when the fraction of Americans working in manufacturing was near 20%, a delphic study predicted that such employment would dip to 12% by the end of the century. Alarmist stories in our newspapers over the past decade seem to bear out this predicted trend.
One advantage of computers in making things is the way they can control actual machines very precisely, so much so that manus no longer has an advantage. Machines do their jobs uniformly so that a constant level of quality can be maintained. Computers are rather single-minded, ready to send the right commands to a machine to do just what a design engineer had in mind, or at least what he told the computer to do.
The greater influence of automation has been on the design engineer's own job, however. Computers are now being used to design and test products electronically. Data are transferred from department to department much faster, eliminating delay, duplication, and the inevitable associated errors. Designs may now be tested in computers and parts that would interfere modified, thus eliminating full stages of costly mock-ups. New designs are being done as modifications of existing designs, with great savings in unnecessary duplication. Designs are being simplified for more efficient manufacturing, thus improving their reliability. Finally, the speed that automation has allowed gives manufacturing the increased nimbleness to produce small lots of goods efficiently and to begin making customized products in large factories.
The result of all this is that employment is dropping not because industry is failing but because it is so much better that it needs fewer workers. Its products have become cheaper and better.
2. Automation in Astronomy
At Tennessee State University we are convinced that automation is the future of observational astronomy. We have gained this perspective from our experience with automatic photometric telescopes (see Fig. 1) and with using computers to plan observing and analysis of observational data.
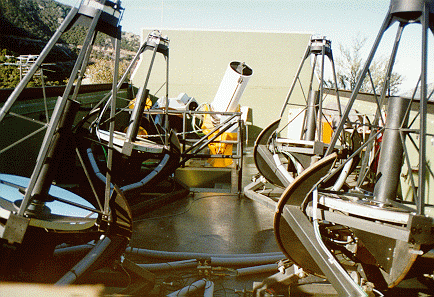
Figure 1. The future of observational astronomy. Shown is the APT farm managed by Lou Boyd atop Mt Hopkins in Arizona. Each of these telescopes observes automatically, under computer control, usually with nobody on site. TSU manages four of the present eight telescopes in this facility. Others are managed by principal astronomers at Villanova, Franklin and Marshall, the College of Charleston, and Arizona State.
The traditional arguments in favor of manual observing--that it's romantic to go to the observatory and that manual observing gives better control of results and higher quality--lose their vigor in the cold light of experience. Ignoring the romance of Luddism, we find automation has made our photometric observing both cheaper and a lot more effective. Thus the reasons for automating astronomical observing are exactly the same as for manufacturing. We break down these advantages as follows:
1. Increased Efficiency: Data can be gathered more economically by automatic robots than by manually controlled machines.
a. Individual observations are less expensive. Although allocation of expenses to particular projects is subject to criticism, the cost per observation does seem considerably less with robotic telescopes. Henry (see ``The Fairborn/TSU Robotic Telescopes Operations Model," this volume) has compared costs of observing trips he and I made to Kitt Peak in the early 1980's with routine observing with APT's. He finds the manual observing was about twenty times as expensive per differential photometric observation of a variable star. These dramatic savings were realized in time not spent at the telescope as well as in the large amounts of time spent reducing data that had to be manually corrected for the inadequate data logging system used at Kitt Peak.
b. More data can be gathered per star. Automatic telescopes can observe during all clear weather available. Manual observers rarely do, even if they have the stamina to try. Thus with robotic telescopes analyses are less likely to be based on an inadequate number of data.
c. Astronomer's time is used more effectively; in planning observations and analyzing data. Given current salaries and overhead rates, an astronomer's time is worth about $50 per hour. This is too valuable a quantity to waste on such repetitive tasks as manual observing.
2. Increased Quality: The same qualities of automation that make it efficient also make it effective.
a. All data are gathered in the same way. This is especially important, since it means that quality can be defined and causes for degradation identified. Quality is thereby improved incrementally with elimination of a succession of problems (see Henry, ``The Development of Precision Robotic Photometry," this volume ).
b. Planning for quality is easier and indeed necessary. Automated observing places much more rigorous demands on equipment than does manual observing. Automated instruments must be strong enough to stand up to mind-numbing repetition. There will be no human observer to fix them when they break or to compensate for their maladjustments. Such machines must therefore be designed so they won't break in the many ways that manual observers have traditionally been expected to tolerate. When robotic telescopes do break, they must be easy to diagnose and to fix, as well. Furthermore, an automated observing program must be designed with the same attention to quality. It must incorporate a strategy for obtaining routine calibration data and quality-control observations. These ancillary data then form a database with which the stability of the instrument can be assessed and incremental improvements planned. Such planning is very difficult in the typical Balkanised manual observing at communal facilities.
c. Different kinds of observing programs can be attempted. Traditional observing has been based on short observing runs lasting from several nights to several weeks. Most professional astronomers cannot afford the living expenses to use more than a few of these sessions each year. The result is that projects requiring long strings of data cannot be done effectively, and astronomers are effectively limited to projects that do not require long-term monitoring or amassing huge numbers of observations. Automation is not subject to such constraints, and this makes a whole new group of studies possible.
3. Automating Spectroscopy
The astronomical research we do at TSU concentrates on magnetism in cool stars. Specific projects are monitoring highly spotted variable stars, discovery of new variable stars, determining times of minimum for eclipsing binaries, interpreting eclipses of spotted (RS CVn) stars, monitoring semiregular variable stars, constructing chromospheric models for giant and supergiant stars, and defining the subtle luminosity changes in old Sun-like stars. Many of these are based primarily on photometry from APT's. However, most depend on using combinations of photometry and spectroscopy, with spectroscopy becoming increasingly important to us because spectra contain so much more information than individual photometric data. Our experience teaches that a combination of very efficient photometry from APT's with inefficient spectroscopy from manual observing runs at the National Observatories is quite limiting. We therefore yearn to do the spectroscopic parts of our research as efficiently as the photometry by building and managing an automatic spectroscopic telescope (AST). To preserve the simplicity required for reliability, such a telescope would necessarily be tailored to our research interests.
4. A Research Program for an Automatic Spectroscopic Telescope
We have identified a group of projects that form the basis of an observing program for an AST. This group includes a wide range of monitoring projects that would be either impracticable or prohibitively expensive when done with manual observing, several large-scale surveys of cool stars, and projects that improve the way data are taken and interpreted. These potential research projects are the following:
1. Search for magnetic cycles in moderately to highly chromospherically active stars. This program consists of monitoring stars with spectra from AST's at H-alpha and Ca II to determine how the line emission tracing chromospheric activity changes with time. Whether such chromospheric emission can change is unclear, but Dorren & Guinan (1990) have claimed an effect in HR 1099. Such spectroscopic monitoring is even more impracticable with manual observing than is the photometric monitoring.
2. Routine Doppler images of spotted stars. We still do not know what changes in the mean brightness of stars like RS CVn mean in terms of magnetic cycles. Most of these highly active systems at any one time have two dark regions on their surfaces, which we call spots, and which persist for roughly two years each (e.g., Henry et al. 1995). They seem to be either persisting groups of moderately large spots or groupings of spots that just seem to persist. We intend to determine what causes the changes in effects of these spot groups by combining spectroscopy with photometry to define the changing spot distributions in a group of about five spotted stars. The advantage of an AST lies in the vastly better data base it can amass, which would allow both excellent phase coverage at individual epochs as well as several independent analyses for a given year.
3. Variability of chromospheres in cool giant stars. Little is known about how the chromospheric structures of cool giants, the ones with large mass loss in winds, change with time. Do they have magnetic cycles? Are there changes related to pulsation of the star? If so, there would be hints about the chromospheric heating. We are obtaining exploratory observations in this area at NSO, but few stars can be studied in this way.
4. Winds of cool giant stars. Properties of winds in cool (G-M) giants are based primarily on a few observations of a moderate number of stars. We do not really know how winds in these stars change with time and what relation any such changes have to variations in chromospheric or photospheric structure. Many observations, over long timescales, are required to answer this question.
5. Pulsations in SR variables and long-period variables. These are M giants that pulsate multiperiodically on timescales of a few weeks to 200 days. UV observations show that their chromospheric emission is modulated on pulsational timescales in many cases, raising the possibility that chromospheric heating in these stars comes mostly from long-period waves (e.g., Eaton, Johnson, & Cadmus 1990). Intense monitoring of several in chromospheric lines is needed to explore this possibility.
6. Variation of mass loss in R CrB stars and shell stars. These are stars with high rates of mass loss thought to be passing from the stage of red supergiant to that of planetary nebula. An aspect of this mass loss is the formation of dust clouds that sometimes block our view of the star. It seems exceedingly queer that a star would puff off a cloud of gas or dust. Effects of the clouds appear dramatically in photometry; we are interested in the conditions in winds that lead to their formation, which may appear in spectra of wind lines.
7. Eclipses of zeta Aur binaries. Systems in which a K supergiant eclipses a B dwarf with its chromosphere and wind let us determine in detail properties of chromospheric gas in actual stars. Although UV spectra follow the chromosphere and wind to much greater height (e.g., Eaton & Bell 1994), optical observations may be used to sample the gas in the lower parts of the chromosphere where most of the intrinsic emission lines are formed. In fact, repeated observations of three systems, 31 Cyg, 32 Cyg, and zeta Aur, will be needed to define properties of the lower chromospheres of cool supergiants. AST's promise the flexibility to make these observations practical.
8. Orbits of long-period binaries and triple systems. These stars may be analyzed to provide basic information about stellar masses and evolution. This is especially important for combination with measurements of these same stars from the coming generation of powerful interferometers.
9. Mass-exchange events in Algol binaries. These systems transfer mass from a less massive to a more massive component through Roche-lobe overflow. The mass exchange seems episodic and elusive, hence difficult to detect with manual observing. Statistical studies requiring many spectra over long periods of time will determine the rate of evolution in Algol systems.
10. Mass ratios of Algol binaries. Doppler images of these systems will give a measure of the sizes of the cool, mass-losing star, hence of the mass ratio of the system (e.g., Eaton, Hall, & Honeycutt 1991). Such information is needed to determine accurately the evolutionary stage of the system and to determine accurate masses and other properties of the stars for theoretical simulations.
11. Survey of H-alpha in all bright cool stars. H-alpha is a probe of the upper chromosphere in cool stars. Variations of it from star to star give an indication of the chromospheric variation expected in a given star. We have observed a significant fraction of the cool giants brighter than V=6 with the McMath-Pierce solar telescope (Eaton 1995). We intend to survey all the cool giants within reach of our telescope to determine how chromospheric absorption depends on metallicity as well as on basic properties of the star.
12. Discovery of more stars with composite spectra. These would be discovered by comparing H-alpha and violet spectra of a great many stars. They will be used to discover more long-period binaries and to assess differences in the chromospheric activity of single stars and binaries.
13. Survey of Li strengths (abundances) in cool giant stars. This is to tell better how Li abundance changes with evolutionary stage. Since Li depletion is still rather poorly understood theoretically, especially in binaries, an assay of as many stars as possible is needed to understand fully what abundances are really encountered under different conditions.
14. Determination of how to obtain more precise routine spectra. In order to accomplish our scientific goals, we must figure out just how to control and characterize the instrument to give reliable observations routinely. We have done this to dramatic effect with photometry. Doing so with spectra would vastly improve the quality of observational results and conclusions.
15. More efficient analysis of spectra. The data rate of an AST will force analysis programs to become more efficient in the way spectra are used. Thus an automatic spectroscopic telescope has the potential to revolutionize the way data are analyzed and interpreted and the results published.
Acknowledgments. I would like to acknowledge numerous helpful discussions of the ideas in this paper with Gregory W. Henry. This research has been supported in part by NSF grant HRD 9104484.
References
Dorren, J. D., & Guinan, E. F. 1990, ApJ, 348, 703
Eaton, J. A. 1995, AJ, 109, 1856
Eaton, J. A., Hall, D. S., & Honeycutt, R. K. 1991, ApJ, 376, 289
Eaton, J. A., Johnson, H. R., & Cadmus, R. R. 1990, ApJ, 364, 259
Eaton, J. A., & Bell, C. 1994, AJ, 108, 2276
Eaton, J. A., Henry, G. W., Bell, C., & Okorogu, A. 1993, AJ, 106, 1181
Henry, G. W., Eaton, J. A., Hamer, J. W., & Hall, D. S. 1995, ApJS, 97, 513